Overview and key points:
Sulfur is an element which the body uses for sulfation reactions.
Sulfur can be acquired in many forms such as:
Inorganic sulfate, sulfite and hydrogen sulfide found in various foods and sources of water
Dietary organosulfur compounds: Amino acids found in proteins (methionine, cysteine, taurine) & sulfur compounds found in brassica vegetables and alliums (glucosinolates, sulforaphane, isothiocyanates)
Various forms of sulfur need to be converted into inorganic sulfate before they can be used in sulfation
Inorganic sulfate reacts with ATP (energy) to form “activated sulfate” known as PAPS, and PAPS is used for sulfation
Sulfation is needed for many important processes including phase two liver detoxification and the synthesis of macromolecules such as glycosaminoglycans and digestive secretions
Glycosaminoglycans are carbohydrate chains associated with cell membranes. They play important roles in providing structure to the cell and extracellular domain, cell-cell communication, and transportation (amongst other things)
Glycosaminoglycans possess a strong negative charge which allows them to interact with biological water in a unique way, forming a “gel-like” phase
The majority of sulfur is obtained via dietary amino acids, which must undergo several reactions (such as sulfoxidation) to become sulfite and subsequently sulfate
Hydrogen sulfide gas is used as a signalling molecule for various purposes, but can also be utilised for the production of sulfate. This gas can be synthesised both in human tissue and by bacteria populating the gastrointestinal tract
Various forms of bacteria can produce hydrogen sulfide gas from different sulfur sources: sulfate-reducing bacteria can convert inorganic sulfate/sulfite, whereas other species such as E.Coli, H.Pylori, and Clostridia are capable of metabolising cysteine
Full article
Sulfur is an element found in the earth’s crust, and is the eighth most abundant mineral in the human body. It is essential, which means that humans are unable to synthesise it, and so it must therefore be acquired through dietary means. Sulfur occurs in several different oxidation states, such as sulfide, sulfane, sulfite, and sulfate. The human body mainly utilises sulfur in the form of inorganic “sulfate” (SO4), a highly oxidised compound which is the end-product of sulfur metabolism. This means that any other form of sulfur must first be converted into sulfate before it can be put to biological use.
In the body, sulfur is not found as an isolated element but instead as a compound with other elements – forming organosulfur compounds. There are various classifications of organosulfur compounds, which include thiols/sulfhydryls, isothiocyanates (breakdown products of glucosinolates), disulfides, sulfoxides, sulfides, and many others.
These compounds are found in all living creatures and are used to make up specific amino acids, enzymes and coenzymes, vitamins, and hormones.
Here is a list of some sulfur-containing organic molecules found in the human body:
Biotin, Pantothenine (vitamin B5), Thiamine (vitamin B1)
Acetyl CoA + CoA
Methionine, SAMe, Homocysteine
Cysteine, NAC, Metallothionein
Taurine, Alpha Lipoic Acid, Glutathione
Sulfur (in some form) is found in every human cell and is involved in a vast range of physiological functions such as:
Cellular energy metabolism
Blood glucose control
Secretion of digestive hydrochloric acid and pancreatic enzymes
Antioxidant and detoxification (via sulfation phase II pathway)
Hormone and neurotransmitter synthesis
Immune cell function
Protects integrity of nervous, joint, and vascular tissues
Lipoprotein regulation (LDL, HDL, cholesterol etc)
Forms skin, nails, hair, and maintains mucous membranes
DNA transcription and replication
Dietary and non-dietary sources of sulfur
The body sources sulfur either in an inorganic form or in an organic form. Inorganic intake only accounts for a small proportion and mostly in the form of sulfate, sulfite, or sulfur dioxide. These forms are obtained through drinking water, inhalation, and through the diet.
Most is obtained in the organic form found in plant material, dairy products, eggs and animal flesh as organosulfur compounds (glutathione, amino acids, sulfated glycosaminoglycans). Dietary proteins rich in sulfur-containing amino acids methionine, cysteine, and taurine, are the primary sources. Certain vegetables are also very high in organosulfur compounds.
Here is a list of sulfur-rich foods:
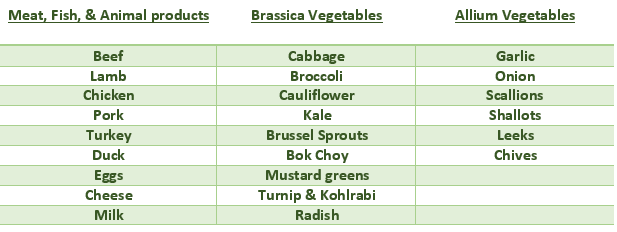
Homocysteine, another intermediary amino acid derived from methionine and involved in the methylation cycle, can also be metabolised to provide sulfate. In addition to this, other sources of organic sulfur may be derived from non-dietary sources. For example, gut bacteria are able to metabolise colonic sulfomucins to liberate sulfur compounds. Furthermore, the gut bacteria can also de-conjugate sulfated bile acids.
A variety of nutritional supplements also provide a rich source of sulfur. These include: glutathione, n-acetylcysteine, alpha lipoic acid, glucosamine sulfate, chondroitin sulfate, methylsulfonyl-methane, indole-3-carbinol, di-indolemethane, milth thistle taurine.
Why is Sulfate important?
The main goal of sulfur metabolism is to produce inorganic sulfate. The body will take all other kinds of sulfur (and there are MANY) and shove them through a whole host of complex biochemical processes just to convert them to sulfate. Why does it do this?
Well, it turns out that inorganic sulfate has some rather extraordinary properties and is essential for a process called sulfation to occur. Sulfation refers to the conjugation (aka transfer) of a sulfur group from a donor molecule to another molecule (a reaction which is catalysed by sulfotransferase enzymes). The donor molecule in these reactions is usually 3′-phosphoadenosine-5′-phosphosulfate (PAPS), otherwise known as activated sulfate.
Inorganic sulfate is necessary for the biosynthesis of PAPS. The reaction requires inorganic sulfate and adenosine triphosphate (ATP), and is catalysed by the enzyme PAPS-synthetase. Simply understand that sulfation means transferring and attaching a sulfate from one place to another place, and it requires activated PAPS to do this. Newly synthesised PAPS can then beutilised and transferred elsewhere via the sulfotransferase enzymes (SULT).
Sulfation is an important route for detoxification, where sulfate is conjugated with many drugs, hormones, neurotransmitters, xenobiotics and toxic substances, so that they can be eliminated safely. Sulfate can be also attached to cholesterol to make it more hydrophilic, and therefore more 'transportable' via circulation.
Additionally, sulfation is involved in the synthesis of macromolecules such as mucins, cholecystokinin, gastrin and the glycosaminoglycans.
Glycosaminoglycans (GAGs) are long, unbranched carbohydrate chains that exist in association with cell membranes and which protrude into the extracellular space. During their synthesis, sulfate is donated by PAPS to become incorporated into the glycosaminoglycan chains to produce sulfated-GAGs. It is important to note that sulfate is the rate-limiting factor in both PAPS and GAG synthesis, meaning that sulfate insufficiency will reduce the synthesis of both products.
Sulfated GAGs are special in that they maintain a highly negative charge on the surface of the cell, and have the ability to bind with large quantities of water to form a “gel-like” substance which makes up extracellular matrix- the portion outside of cells.
Here are some examples of GAGs:
- Chondroitin sulfate: The most common GAGs and located in ligaments, cartilage, and tendons.
- Heparan sulfate: Attached to almost all cell surfaces and found in the basement membrane of epithelial tissues
- Dermatan sulfate: Located in the vasculature, the skin and the cardiac valves.
- Keratin sulfate: Located in the loose connective tissues in partnership with chondroitin.
- Heparin: A component of mast cells, found in the liver, skin and in the lungs.
Some basic functions GAGs
Structural component of the extracellular matrix
Cell signalling and adhesion
Facilitate hydration of tissues
Act as lubricants and shock absorbers to support compressibility of cartilage
Transport/movement of nutrients and wastes
Tissue formation
Cell migration and movement
Many roads all lead to SULFATE
It has already been stated that the body is required to oxidise all other forms of sulfur into the end product – sulfate - so that it can be utilised in the sulfation pathway. A small amount of inorganic sulfate is absorbed through digestion, however the large majority comes in an organic form, which means it needs converting first.
Below, I will briefly outline some of the main ways by which the body does this.
Sulfoxidation
Gastrointestinal absorption of organic sulfur-containing amino acids (cysteine and methionine) is relatively high. These free amino acids yielded from digestion rapidly enter circulation and become available to tissues throughout the body for several purposes. They can be incorporated into new proteins during protein synthesis, they can be directly oxidised for energy, and they can be metabolised for the production of the sulfur-antioxidant glutathione.
Additionally, cysteine can be oxidised into sulfate to be utilised for sulfation. Methionine, on the other hand, cannot be oxidised into sulfate. Thankfully, though, methionine can be converted into cysteine.
Dietary methionine undergoes a sequence of reactions catalysed by various enzymes to be converted into cysteine. These processes fall under the categories of “methylation” and “transulfuration”.
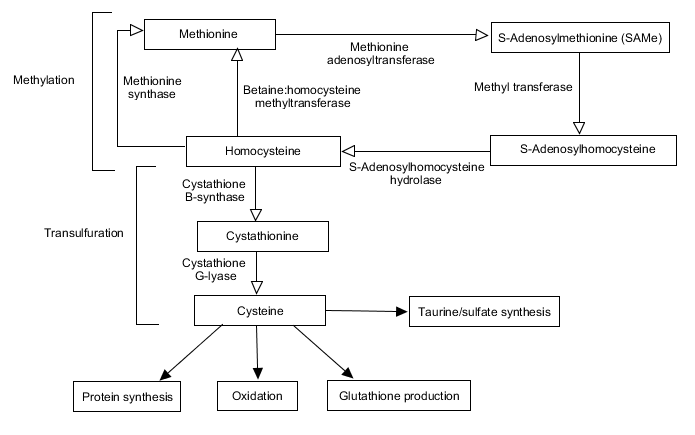
Here is a brief overview of the process:
Methione is methylated into S-Adenosylmethionine (SAMe)
SAMe is converted into S-Adenosylhomocysteine (SAH)
SAH finally converts to homocysteine
Homocysteine gets converted into Cystathionine by the enzyme Cystathionine-Beta-Synthase (CBS)
Finally Cystathionine is further converted into Cysteine via the enzyme Cystathionin-Gamma-Lyase (CGL)
The next stage involves the transformation of cysteine into sulfite via several steps.
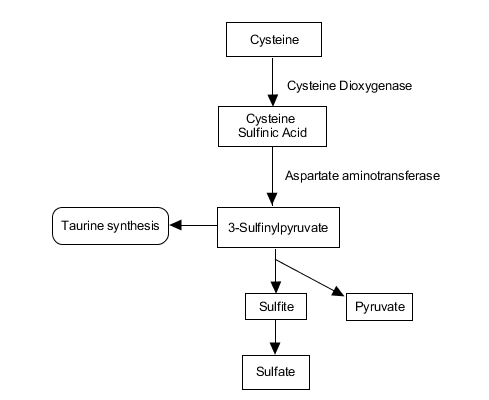
Cysteine is oxidised to cysteine-sulfinic acid ( aka 3-sulfo-alanine) by Cysteine Dioxygenase
Cysteine-sulfinic-acid is transaminated by Aspartate-Transaminase to form 3-Sulfinyl-Pyruvic Acid.
3-Sulfinyl-Pyruvic Acid can then spontaneously react to produce pyruvate and sulfite
Sulfite
Sulfite is an intermediary metabolite in sulfoxidation (and sulfate production). Unfortunately, sulfite is a toxic substance capable of inducing inflammation and exerting damage to cellular components such as DNA and proteins. Sulfite also can deplete antioxidants such as glutathione and reduce cellular energy (ATP). Hence, sulfite must be kept at relatively low quantities and rapidly detoxified.
The body does this via an enzyme called sulfite oxidase (SUOX) which is located in the mitochondria of cells (in very high quantities in the liver and lung cells).
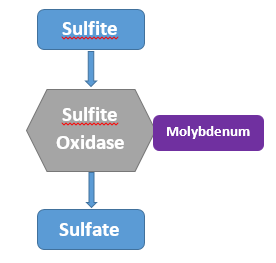
Sulfite oxidase is responsible for oxidising sulfite into sulfate, the form that the body can utilise. It is important to note that this enzyme requires the trace element molybdenum to function. People who are insufficient in molybdenum (for whatever reason) may experience negative reactions when they consume sulfites or excess sulfur-containing foods. Glyphosate is a known chelator of molybdenum, and therefore can contribute to sulfite oxidase dysfunction. Furthermore, heavy metals such aluminium and mercury can also interfere with the enzyme's function.
Once inorganic sulfate has been made available, it can further be activated by PAPSS to produce PAPS, and can enter into one of the many pathways involved in sulfation.
Hydrogen Sulfide – another source of sulfate
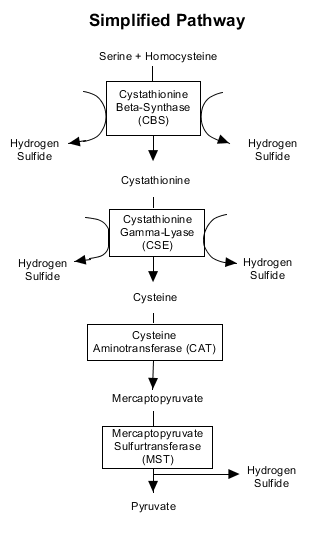
The metabolism of homocysteine (transulfuration) not only provides cysteine (and subsequently sulfite), but also yields a gas known as Hydrogen Sulfide (H2S). The three hydrogen sulfide producing enzymes are cystathionine-gamma-lyase andcystathionine-beta-synthase, both of which utilise B6 as a cofactor, and 3-mercaptopyruvate sulfurtransferase which uses zinc as a cofactor. It can be synthesised in many tissues throughout the body, varying from the central nervous system, liver, kidney, cardiovascular system, lungs and within the gastrointestinal tract.
Dr Stephanie Seneff has described this gas as being like a “ghost” because of its ability to travel through tissues very easily via simple diffusion (it does not require a “transport system”).
Hydrogen sulfide has historically been studied as a toxic gas and environmental pollutant. However, in recent years research has shown H2S to be a highly active “gasotransmitter”. Physiological roles of H2S include blood vessel relaxation, neuromodulation, angiogenesis, regulation of the inflammatory response, insulin release, energy metabolism, and cardioprotection.
Due to the fact that this gas contains inorganic sulfur, it can be oxidised as a source of sulfate under certain conditions by the action of several enzymes.
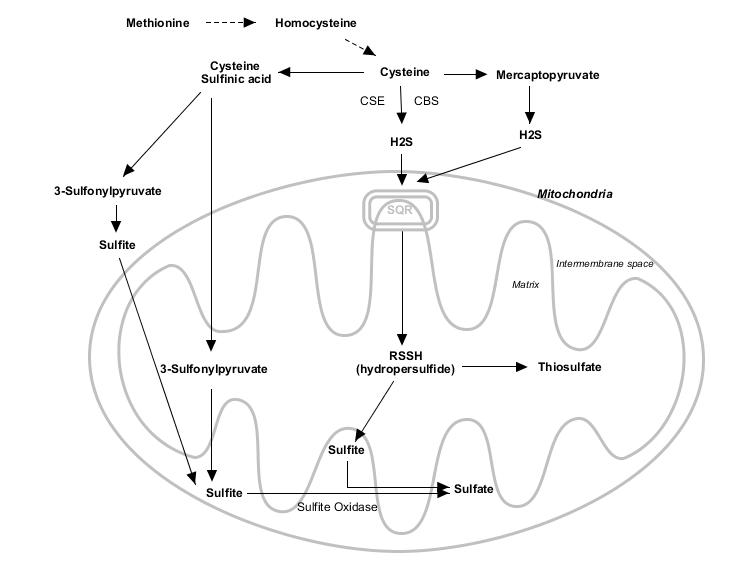
As can be seen from the above diagram, H2S can be rapidly oxidised in the mitochondria by an enzyme called “sulfide-quinone reductase" to produce either thiosulfate or sulfite, both of which can be further oxidised into sulfate.
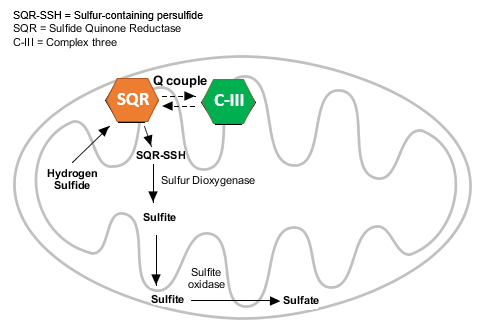
Sulfate-reducing bacteria and fellow microbial metabolism
Certain species of bacteria that populate the gastrointestinal tract have the ability to convert various forms of sulfur into hydrogen sulfide gas.
Species falling under the category of “sulfate-reducing bacteria” (SRB) living in the gut utilise inorganic sulfate as the terminal electron acceptor in respiration. The dominant genera are called “Desulfovibrio”, “Desulfobulbus” and “Desulfotamaculu”. These bacteria are “hydrogenotrophic”, which means that they like to consume hydrogen as part of metabolism. This is because they require an electron donor such as hydrogen, however they can also use other electron donors like lactate or formate. The end product of sulfate metabolism in this context is hydrogen sulfide gas.
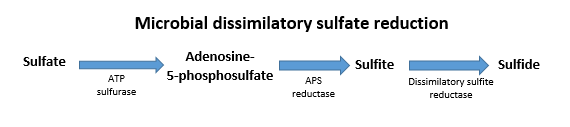
The source of inorganic sulfate can either be derived from external sources such as the diet and drinking water, or it can be extracted from broken down components of the intestinal cells themselves. Through the use of sulfatase enzymes, these bacteria and others are able to liberate sulfate by breaking down sulfomucins and mucopolysaccharides (cell constituents) which line the intestinal tract.
Furthermore, these bacteria (and others) are also able to produce hydrogen sulfide gas from its significantly more toxic counterpart, sulfite, via the sulfite reductase enzyme.
Species such as E.coli, H.pylori, Salmonella, Clostridia, Streptococcus, and Enterobacter can also convert the dietary amino acid cysteine into hydrogen sulfide via the enzyme cysteine desulfhydrase.
Hydrogen sulfide produced in the gut may be directly absorbed into circulation and diffuse through tissue to the liver, pancreas, and spinal collumn, or it may be oxidised into thiosulfate/sulfate by cells in the colon (via SQR) and subsequently utilised in those forms.
コメント