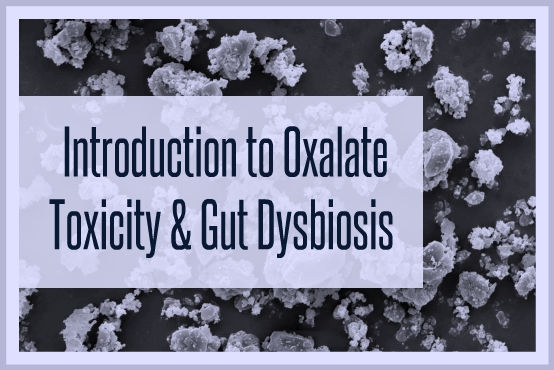
Still suffering from bloating, gas, constipation, diarrhoea, or abdominal pain? Eating lots of "healthy" foods like chia puddings, spinach salads, and kale smoothies? Been through multiple of rounds of antibiotics and antimicrobial herbs, yet don't seem to see any long term improvements? That could be because, for many people, treating small intestinal bacterial overgrowth (SIBO) is not as simple as:
"kill off the bad guys and replace them with the good guys"
In the previous article of this series - Sulfate IV: Chronic SIBO/Gut Dysbiosis As A Protective Adaptation To Supply Sulfate - I postulated, based on the work of Dr Stephanie Seneff and co, that long-term recurrent gut dysbiosis (or hydrogen sulfide-dominant SIBO) may actually play beneficial roles for individuals who have defective sulfur metabolism. It is theoretically possible that the excess production of hydrogen sulfide gas may help to replenish or transport sulfur via a novel alternative route when no other options are available. I speculated that changes in the bacterial flora populating the gut were perhaps adaptive to promote host health, and that blindly destroying the gut flora with antimicrobials/antibiotics may not be the most suitable long-term, sustainable option.
Therefore, SIBO may not actually be a specific disease entity in and of itself, but rather a potential solution to an underlying deficit. In the previous article, I explained that glyphosate - Monsanto’s infamous herbicide “Roundup” - was implicated in defective sulfur transport and utilisation.
Here, I am going to write about another factor which has also been shown to significantly disrupt sulfur chemistry and gut homeostasis, and which doesn’t receive a fraction of the attention it deserves. I am going to talk about oxalate.
First of all, I would like to make clear that I am by no means an expert in this field and it is only quite recently that I have begun to learn about this topic in more depth. Much of the information shared in this article has been derived from the extensive work of Susan Owens and members of group that she runs on facebook – Trying Low Oxalates, and the website Low Oxalate Info.
It is important to note that the information in the article does not even scratch the surface, and that oxalate metabolism is a highly complex and multifaceted aspect of physiology. However, I have tried my best to compile the main points and explain concepts in a way that should hopefully be understandable. That said, I highly recommend anyone seeking further information to head over to that group, or scroll down to the bottom of the page for some further resources.
What is oxalate/oxalic acid, and where does it come from?
Oxalic acid is a corrosive organic acid which has a high affinity for binding with various minerals such as calcium, sodium and potassium. Upon binding with mineral ions oxalic acid becomes an oxalate salt. These salts may occur as either water-soluble forms like sodium oxalate and potassium oxalate, or alternatively they can occur as insoluble salt such as calcium oxalate.
Oxalate is a crystalline compound which is ubiquitous in nature being a metabolic end-product of plant physiology. The most abundant form found in nature is calcium oxalate, which is common amongst many families of plant. However, small amounts of oxalate are also a product of normal human metabolism.
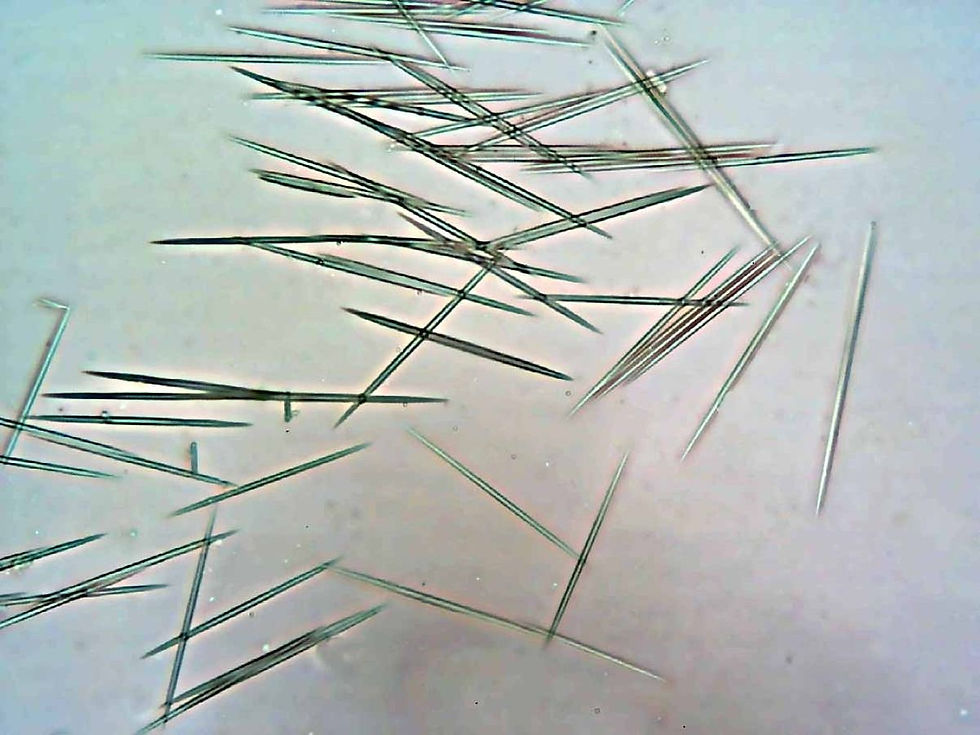
These crystals boast an intimidating structure, where they can exist in spikey formations, sharp irregular rectangles, and long fork-like needles (as shown below). Unsurprisingly, oxalates can cause significant physical damage to exposed tissues and cells. Because of this, researchers have long proposed oxalate to be a tool employed by plant as an built-in defense mechanism to deter hungry herbivores and to promote plant survival.
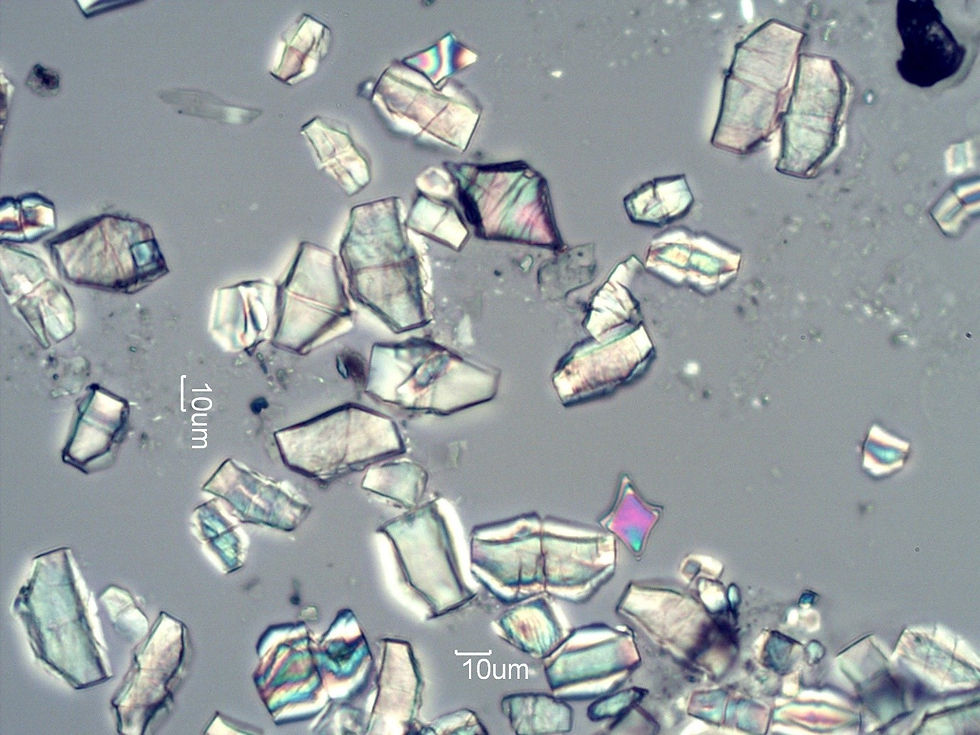
Although oxalate is found in most plant material, the quantity differs greatly between each type of plant. Certain plants such as amaranth, sweet potato, buckwheat, cacao, nuts, spinach, rhubarb, beetroot, kale, kiwi, and collard greens contain extremely high levels of oxalate. On the other hand, many other plants contain negligible levels of oxalate, and as a general rule animal products contain the least amount of oxalate.
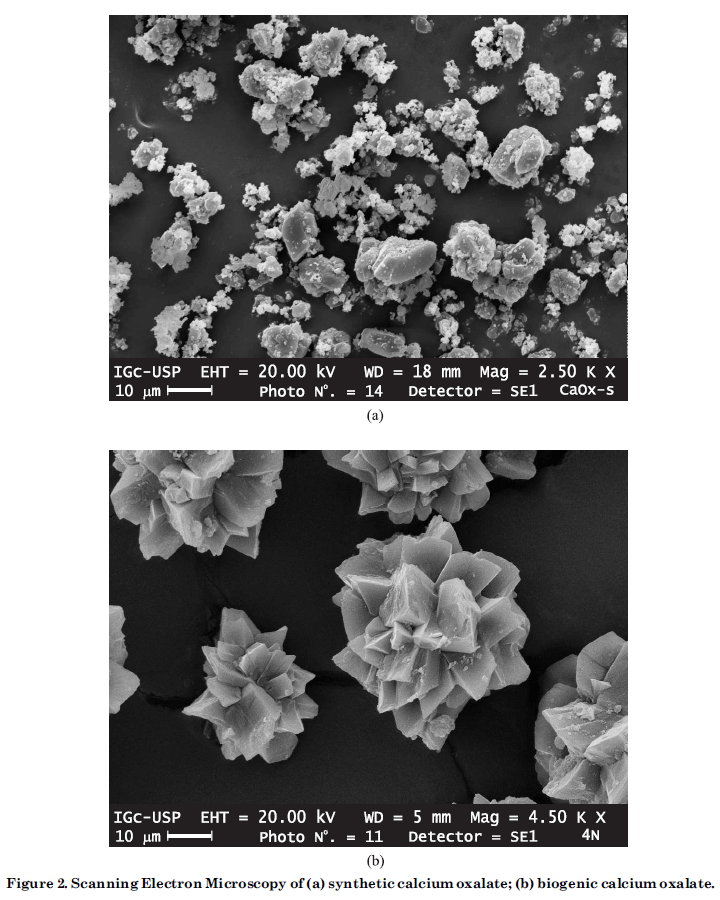
When humans consume oxalate-containing foods, the entry route into the body begins in the gut, and the degree of absorption is believed to depend on the solubility. Water-soluble salts (potassium, sodium oxalate etc) and free oxalate can be readily absorbed into circulation, whilst insoluble forms (calcium and magnesium to a lesser degree) are bound up, unable to pass through the intestinal wall, and are therefore likely to remain within the gastrointestinal tract to later be excreted .
Oxalate has a very high affinity for binding calcium, and so consuming adequate calcium alongside oxalate in a meal can greatly inhibit oxalate absorption. Although whilst this is one benefit, this also means that calcium bioavailability in high oxalate foods is likely very low. Therefore, relying on spinach or almonds for their “high calcium content” is probably not a good idea. Furthermore, poor fat digestion stemming from pancreatic insufficiency, SIBO, or hepatic cholestasis (sluggish bile flow) can cause non-emulsified fatty acids to bind calcium in the gut, resulting in more free oxalate for absorption.
Certain gut microbes such as Eubacterium lentum, Enterococcus faecalis, Lactobacillus sp., Bifidobacterium infantis, and most notably Oxalobacter formigenes, are responsible for degrading oxalate in the intestinal tract. In this way, these microbes are indispensable to the health and stability of the human body. These same microbes are sensitive to specific groups of antibiotics (fluorquinolones, tetracycline, nitroforatoin etc), and are therefore likely to be detrimentally affected by antibiotic exposure.
Following the absorption of the soluble oxalate into circulation, it is eventually expelled via the urine through the kidneys. The kidney is the primary route of oxalate excretion, and can only cope within certain limits.
When oxalate levels rise and outweigh the kidney’s ability for excretion, this can lead to the deposition of insoluble oxalate crystals in various organs and tissues. The most well-known and researched consequence of this is the development of kidney stones. Around 80% of kidney stone cases are characterised by calcium oxalate.
In medical terminology, high oxalate body-burden and elevated urinary oxalate excretion is referred to as “Hyperoxaluria”. Hyperoxaluria can exist in multiple forms. Primary hyperoxaluria is characterised by inherited genetic errors involving the enzymes responsible for metabolising oxalate precursors (AGXT, GPHPR, & HOGA1).
In contrast, secondary hyperoxaluria is caused by increased dietary oxalate, enhanced intestinal absorption (enteric hyperoxularia) due to altered gut microflora or defective digestion of lipids, or high intakes of oxalate precursors (glycine, vitamin C, ethylene glycol).
How oxalate affects the human body
As previously mentioned, small amounts of oxalate is a normal part of normal human physiology. However, when in excess it becomes a major issue. Oxalate can travel via circulation and deposit to form crystals in various tissues including:
Kidney & Urinary Tract
Coronary arteries & Myocardium
Thyroid gland & Spleen
Lymph Nodes & Testis
Intestine & Bone
Eyes & Skin
Liver & Brain
After deposition, oxalate mechanically shreds the surrounding tissues. However, not only does oxalate deposit in tissues, but micron and nano-sized crystals can even enter into the cells. Once inside the cell, oxalate progressively wreaks biochemical havoc:
Apoptotic and necrotic cell death
Ruptures cell membranes and leads to cell swelling
Lysosomal rupture
Cell and nuclear shrinkage
Destruction of cellular organelles
Make no mistake: Oxalate is a poison. It destroys mitochondrial function, alters redox homeostasis via depleting intracellular antioxidants such as glutathione and superoxide dismutase, disrupts the mitochondrial membrane potential and halts ATP (energy) synthesis.
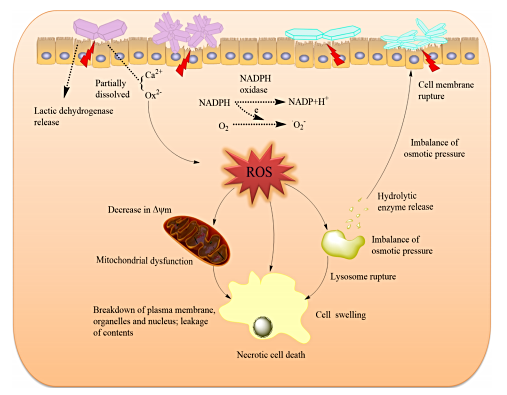
Oxalate also blocks the action of several enzymes involved in energy metabolism such as pyruvate kinase , and the biotin-dependent enzymes pyruvate carboxylase and transcarboxylase.
Furthermore, oxalate has been shown to trigger the activation of the NLRP-3 inflammasome – a multicomponent piece of machinery inside the cell responsible for danger-signalling, cellular defense, and which contributes to long-term, chronic inflammatory processes.

Once insoluble oxalate crystals deposit in tissues, local irritation and inflammation ensues. This can manifest in practically any way, but which frequently includes joint pain, muscle pain, or bladder irritation. The mechanical and biochemical onslaught inflicted by oxalate likely renders local tissue more susceptible to opportunistic infection by pathogenic microorganisms.
Chronic urinary tract infections which are unresponsive to antibiotics are a significant point of interest here. One study demonstrated that E.coli, the most common form of bacteria implicated in UTIs, can selectively aggregate on and around calcium oxalate crystals. A variety of other bacteria including Pseudomonas and Gardnerella have also been detected in urinary oxalate stones. In the context of urinary tract infections, natural prevention and treatment methods have involved the use of urinary alkalizers such as potassium and sodium citrate salts. Alkalizers have been found to be very effective in reducing UTI symptoms, and were also effective at eliminating urinary candidiasis.
Although scientists are not certain about the mechanism by which citrate exerts its effects, the dominant theory is based on that notion that alkalizing the pH of the urine inevitably reduces pain associated with urination. However, it is useful to note that citrate is also effective at solubilising calcium and dissolving calcium oxalate stones. Therefore, if UTIs can be caused by calcium oxalate deposits in the urinary tract, then it would make sense that citrate would be useful as a treatment method.
And so after reviewing the the above points, one can perhaps now understand why oxalate has been implicated in such a wide variety of health conditions which include (but are not limited to):
Kidney stones, & Arthritis
Interstitial cystitis/Bladder pain
Autism & Glaucoma
Inflammatory Bowel Diseases & Coeliac
SIBO
UTI, IC & Vulvodynia
Cystic fibrosis
In the context of oxalate-induced dysfunction or pathology, a large portion of the body's total oxalate load comes in through the diety. However, not all oxalate comes in from dietary sources. In fact, up to half of the total oxalate is actually synthesized by the body itself.
Endogenous oxalate synthesis
Endogenous synthesis of small amounts of oxalate occurs as a normal part of human metabolism. Oxalate can be generated in many types of cells, such as erythrocytes, although the main production site is in the liver. This process is referred to as the “glyoxalate pathway” and is shown below:
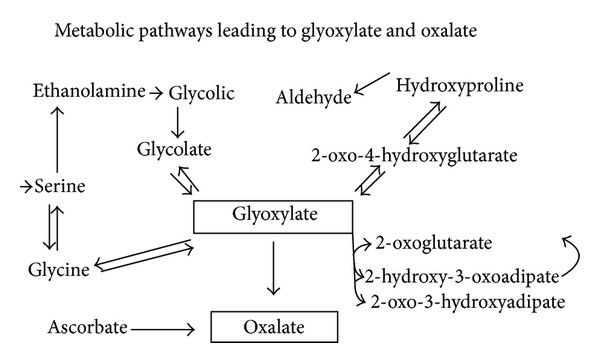
As can be seen above, a compound called glyoxylate is the main endogenous precursor for oxalate. However, ascorbate (vitamin C) is also another important source. Glyoxylate can come from multiple sources. It can be derived from glycolate, or can also be derived from the amino acids hydroxyproline and glycine, although these pathways are thought to be minor under ordinary conditions (more on this below).
Glyoxal, a glyoxylate precursor, can be generated from fats, carbohydrates, and proteins. These mechanisms involve the autooxidation of carbohydrates (including glucose, mannitol, fructose, ribose), the degradation of glycated proteins, and the generation of lipid peroxides. The formation of glyoxal increases under oxidative stress, which indicates that any underlying chronic condition characterised by excessive oxidation may predispose one to oxalate-related problems.
The pool of glyoxylate can then go on to become oxalate, although in a healthy and nutritionally-sufficient system, glyoxylate still has several potential fates. At this point it is important to understand that oxalate is toxic to the human body, and so oxalate synthesis is the “last resort”.
So under the healthy conditions, glyoxylate can be converted back into the amino acid hydroxyproline. Alternatively, it can also be transaminated back into glycine. These are the safest routes, but this conversion is facilitated by the liver enzyme alanine-glyoxylate-aminotransferase (AGT), utilising vitamin B6 (PLP) as a cofactor.
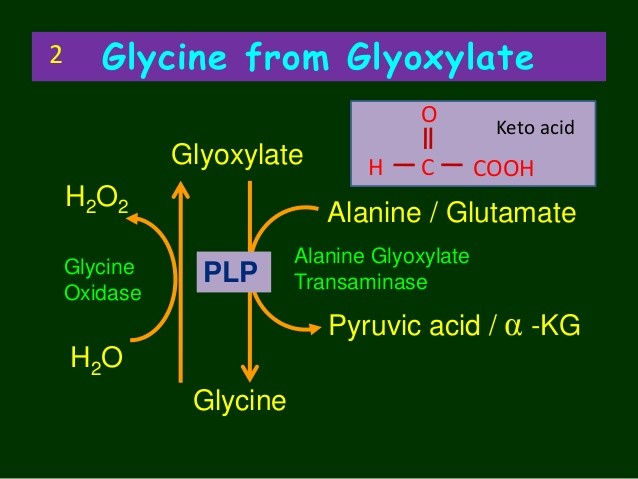
This means that each time glyoxylate needs to be converted into glycine, the liver uses up vitamin B6. This also means that under conditions of prolonged oxidative stress, the excessive processing of glyoxylate is going to increase the requirement for B6. If this goes on for long enough, then B6 can become depleted.
Vitamin B6 depletion inhibits the AGT enzyme , and glyoxylate is instead shunted down to produce higher levels of oxalate. The end results is lower pools of glycine, hydroxyproline, and elevated urinary oxalate.
An important side note:
One might think that simply supplementing with extra glycine or hydroxyproline could be helpful to counteract the deficit, but they would be wrong in this situation.
Under B6 deficiency, glycine supplementation can actually increase endogenous oxalate synthesis. Another study also showed a 7.56-fold increase in endogenous oxalate synthesis when B6 deficient rats were supplemented with hydroxyproline.
Hence, vitamin B6 status plays a key role in maintaining healthy glyoxylate metabolism, and a deficiency (from any known cause) can independently lead to oxalate-related issues.
Although there are many factors which have been shown to deplete vitamin B6, tissue-specific inflammation can rapidly increase this process. On the other hand, oxalate's ability to activate the inflammasome and trigger inflammation in tissue is also a key factor involved in B6 depletion.
What this means in basic terms is that vitamin B6 deficiency can cause internal oxalate issues, but oxalates can also cause B6 deficiency. So this conundrum operates like a vicious, self-perpetuating cycle. Additionally, thiamine deficiency can also increase one's susceptibility to the toxic effects of oxalate, though that is a topic for another article.
Leaky gut increases oxalate - oxalate increases leaky gut and dysbiosis
Steathorrea (maldigestion of fat) and elevated intestinal permeability (aka leaky gut) markedly increase absorption of oxalate. This means that anyone suffering with GI-related issues, autoimmune conditions, or established “leaky gut” are likely at a higher risk for oxalate poisoning.
On the other hand, high levels of oxalate may also be a direct cause of leaky gut. One study on kidney epithelial cells showed that calcium oxalate crystals markedly decreased the levels of occludin and zonula occludens-1, reduced transepithelial resistance, impaired the tight junction barrier, and increased paracellular permeability. Since these mechanisms are basically the same as in the intestine, it seems fair to assume that oxalate may also cause leaky gut in a similar way to gliadin (wheat) and other related compounds.
To make matters worse, oxalate can also disrupt the balance of gut bacteria, producing a state referred to as gut dysbiosis. The microbes in the gut which are responsible for degrading oxalate can become overwhelmed by excess dietary oxalate. One study showed significant growth inhibition of oxalobacter formigenes when exposed to high levels of oxalate:
Interestingly, higher than usual concentration of oxalate was found inhibitory to many gut microbes, including Oxalobacter formigenes, a well-characterized OMBS.
We also observed that some of the prominent gut residents to be negatively correlated with the urinary oxalate, even though they have ability to metabolize oxalate. This indicates that above certain concentration, oxalate could be toxic to these common gut inhabitants; similar results have also been observed with respect to Oxalobacter formigenes
This means that eating too many high oxalate foods may kill off the beneficial and protective bacteria, rendering our microbiome unable to effectively cope with the burden.
Furthermore, a similar phenomenon appears to also occur with resident species of yeast such as Candida Albicans. As in humans, oxalate disrupts energy metabolism in Candida, and research shows that exposure to high oxalates can result in a protective shift from a commensal form to a more pathogenic and invasive hyphal/ filamentous form responsible for building dense protective biofilm. This means that targeting the candida may be futile, since it appears to be more of a symptom - rather than the root cause (which might be oxalate).
Oxalate disrupts sulfur metabolism
Elevations in oxalate, whether due to exogenous overconsumption or due to endogenous synthesis driven by vitamin deficiency and oxidative stress, can negatively impact sulfur chemistry in several ways.
First of all, going back to basic sulfur chemistry, many of the enzymes involved in the transfer of sulfur and synthesis of sulfur containing compounds depend on vitamin B6 as a cofactor. What might happen when B6 becomes depleted due to excess oxalate load? The synthesis of sulfur-containing compounds will decrease, and the downstream effects of this will be impaired synthesis of the sGAGs, impaired intracellular antioxidant systems, and higher levels of oxidative stress.
But to make matters worse, excess oxalate can actually lead to a process known as sulfur-wasting, where much of the body’s sulfate is dumped out into the urine. Dr Rostenberg wrote a comprehensive article on how this process takes place. In short, oxalate and sulfate can enter or exit a cell via the same protein (sulfate anion exchanger) – called Sat-1. Sat-1 is located on the membrane of the colon, kidney, and liver.
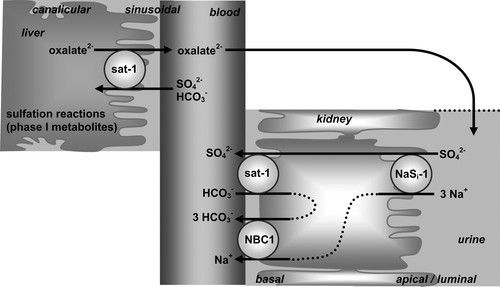
Sulfate and oxalate are bi-directionally exchanged across the membrane, meaning that for every sulfate ion crossing one way, an oxalate passes through the backdoor in the opposite direction. Hence, high amounts of oxalate on one side of a membrane can effectively “pull” large amounts sulfate out from the other side. In the context of kidney filtration, a high body oxalate-burden could theoretically cause us to dump sulfate into the urine whilst retaining oxalate. A similar mechanism may also occur the context of high soluble or free oxalate in the colon. In this way, cells which need sulfur may actually become overburdened with oxalate instead.
In addition to this, elevated levels of oxalate (as would be found in hyperoxaluria) have also been shown to competitively inhibit the uptake of sulfate in cells.
Through disrupting and depleting sulfur chemistry, oxalates can have wide ranging indirect effects on multiple organ systems. To recap, sulfur is essential for:
Sulfated glycosaminoglycans - providing hydration and structural integrity throughout the whole of the body. Lining the vasculature to provide dense negative to facilitate blood flow and prevent blood cell aggregation.
Mucins lining the gut wall, maintaining moisture, fluidity, and integrity of the gut barrier.
Cholesterol sulfate dotted on red blood cells to maintain a healthy zeta-potential.
Sulfated hormones, sulfur-containing compounds and liver detoxification
Therefore, any deficit in sulfate can be deadly... and oxalate potentially has a major role in disrupting this system.
Recall from the previous article in this series, I provided some indirect evidence supporting the notion gut dysbiosis and hydrogen sulfide-dominant SIBO may be potential solutions to a defective sulfate system. I drew upon Stephanie Seneff’s research to lay out a framework whereby certain bacteria (clostridia & sulfate-reducing bacteria) may 'overgrow' on purpose, in a mutually beneficial relationship with the host to replenish much needed sulfur.
Hydrogen sulfide gas was proposed to play a key role in this process. Hydrogen sulfide can be absorbed as a gas from the gut into the blood, and then travel throughout the blood. Furthermore, it can passively enter the cells through diffusing across the cell membranes and bypassing all of the ordinary transport methods. Once inside the cell, it can be oxidised back into sulfite, thiosulfate, and eventually into sulfate which can be put to use.
Given the fact that elevated oxalate can signficantly disrupt sulfur distribution, and perhaps even block the entry of sulfate into cells, it would make sense that the body may intentionally select for changes in the microbiome to provide alternative routes for transport of sulfur in the form of a gas when no other options are available. Although there is not any research to support this speculation, it is interesting to note that poor sulfation is a key feature of autism, and that hyperoxaluria may also play a significant role. At the same time, the sulfate-reducing bacteria such as Desulfovibrio are also found abundantly in autistic children. Do these sulfate-reducers serve their host through providing a utilizable form of sulfur in the face of oxalates and glyphosate? I believe it is possible, and makes intuitive sense... although this is purely speculation on my part.
The unfortunate consequence of having excess hydrogen sulfide in the gut are the many unpleasant side effects and symptoms, and the overproduction of this gas in the gut is generally assumed in both the conventional and alternative medical communities to be something that needs to be "fixed" with antibiotics or antimicrobials.
Conclusion
Based on the above material, I believe that anyone with recurrent SIBO, gut issues, chronic pain, or any of the other associated symptoms or health issue would do well to tho consider oxalate as a potential driving factor underlying their condition. In fact, I think everyone would do well to reconsider their beliefs about so-called "healthy" foods. This especially applies to advocates of a Paleo or ketogenic diet, since the removal of common offending foods are usually replaced with extremely high oxalate foods as part of a staple diet. This includes cacao, teas, almond flour, buckwheat and other gluten-free grains, sweet potato, beetroot, and many other "Paleo" staples.
We need to appreciate that many foods we now consume so frequently were once seasonal, meaning that they would not be available all year round. Furthermore, many of the "superfoods" are obtained from distant tropical countries, and were only introduced to western populations relatively recently in our evolutionary history. Therefore, we should be cautious about whether these novel foods are even tolerable in the long-term. For a more comprehensive overview of this topic, I will direct the reader to Sally K. Norton's article: Lost Seasonality and Overconsumption of Plants: Risking Oxalate Toxicity
Below are some useful resources:
Presentation - AHS17 Lost Seasonality and Overconsumption of Plants: Risking Oxalate Toxicity - Sally Norton
Susan Owens' interview at the Nourishing Hope For Autism Summit
www.lowoxalate.info
https://www.facebook.com/groups/TryingLowOxalates/
Primary and secondary hyperoxaluria: Understanding the enigma
Σχόλια