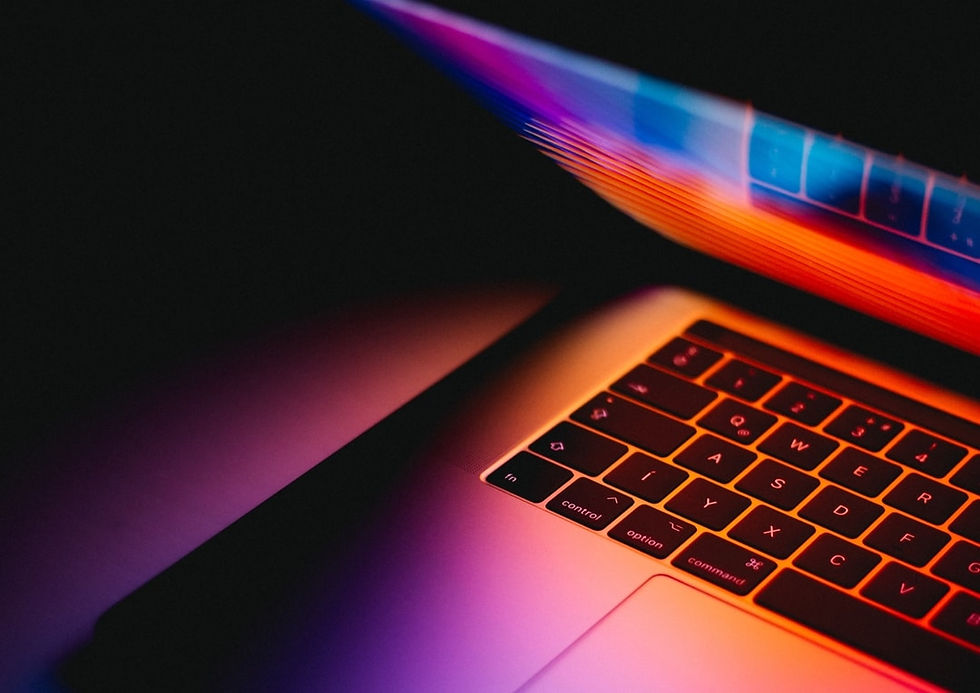
For those who are not aware, electromagnetic hypersensitivity syndrome is characterised by collection of symptoms which manifest when someone comes in close contact with non-native electromagnetic frequencies. Non-native refers to the unnatural, man-made EMF emitted from electronic devices, mobile phones, Wifi, dirty mains electricity, and overhead power lines etc.
The symptoms of EHS are wide and varied in number, but they typically include brain fogginess, insomnia, skin rashes, palpitations, anxiety, depression, physical sensations of pain, headaches and migraines, neuropathies and parasthesias, and burning sensations in the limbs.
Unfortunately, many patients with this condition are dismissed and have their symptoms labelled as purely “psycho-somatic”.
Is EMF-Sensitivity/EHS a real condition?
Despite conventional medical claims that EHS is primarily “psychosomatic”, there have been several researchers who have demonstrated real, physiological changes occurring in EHS. These changes are indicative of increased levels of oxidative stress like those found in multiple chemical sensitivity syndrome. Patients with EHS were found to have increased blood concentrations of malondialdehyde (a lipid peroxide), oxidized glutathione, and nitrotyrosine. Beneficial glutathione-associated biomarkers were lower in 20-40%, and RBC superoxide dismutase and glutathione peroxidase activity were increased in 60% & 19%, respectively. Overall, this trial showed that 80% of EHS sufferers presented with one, two or three elevated biomarkers of oxidative stress [1].
In another study, EHS patients were shown to have higher levels of oxidized antioxidants vitamin E and coenzyme Q10 [2]. RBC glutathione-S-transferase was decreased, along with reduced glutathione. Furthermore, genetic analysis identified two genotypes related to glutathione status [GSTM1 (*0/*0) + GSTT1 (*0/*0)] which were more common in EHS sufferers compared with controls. This specific combination conferred a 9.7 times higher risk of developing EHS, which suggests that some people may be more genetically susceptible to developing this condition than others.
EHS patients were matched with controls in a study measuring cortical excitability parameters using transcranial magnetic stimulation [3]. Those with EHS were found to display significant differences in cognitive and neurobiological activity, demonstrating genuine functional changes in brain function which occur in this condition.
One individual with self-diagnosed EHS was shown to have abnormalities on functional MRI scanning which looked similar to traumatic head injury [4]. Whilst another double-blinded case study demonstrated clear somatic responses to EMF exposure which included muscle twitching, arrhythmia, headache and temporal pain [5].
Some researchers have proposed that EMF-sensitivity may be related to heavy-metal toxicity and exposure. Although one study showed no differences in blood metal concentrations in EHS [6], it is well established that blood levels are not always reflective of tissue metal concentrations. Case studies in Japan correlated dental titanium implants with EHS-related symptoms such as vertigo and dizziness. Interestingly, these symptoms disappeared after the metal implants were removed, leading the researchers to theorize that the titanium was acting as some form of “antennae” for EMF radiation [7].
In addition, the EMF emitted from mobile devices has the capacity to increase the release of mercury vapor from amalgam fillings into saliva [8]. The same effect can also occur through exposure to magnetic resonance imaging (MRI) scanning [9]. Since mercury is a highly neurotoxic substance, it is believed that heavy metal poisoning may be responsible for producing or exacerbating the symptoms associated with EHS.
Based on the above data, coupled with innumerable anecdotes, it would indeed appear that EHS is a genuine physiological condition. This condition appears to feature alterations in brain function, clear differences in biomarkers associated with increased oxidative stress, and somatic responses upon exposure to EMF devices.
However, this does not mean that EHS sufferers are the only ones who are negatively impacted by exposure to nn-EMF. In fact, there is a vast body of literature demonstrating substantial damage done to cellular systems upon exposure to this radiation. To go through all of the evidence would be frankly impossible, and even a comprehensive overview is way beyond the scope of this article.
However, to understand why the addition of specific nutrients may be beneficial in protecting the brain from the negative consequences of EMF exposure, we need to examine some of the processes that occur at the molecular level with irradiation.
Effects of EMF on the brain
The exact mechanisms by which different frequencies of EMF interact with different cell types have not yet been fully characterised. It is thought to involve voltage-gated calcium channel activation and calcium efflux, resulting in elevated nitric oxide synthesis, peroxynitrite free radicals, neuro-inflammatory cascades and glutamate-neuro excitotoxicity. What is clear, however, is that mitochondrial dysfunction and oxidative stress are the main drivers behind EMF-induced brain injury.
The high rate of oxygen consumption and metabolism of the brain, coupled with its high neuronal membrane content of unsaturated fatty acids, make it uniquely susceptible to oxidative damage. This, paired with the fact that mobile devices are generally positioned in close proximity to the face and head, render the brain a prime target for electromagnetic radiation.
Neuro-excitotoxicity is a phenomenon characterised by excessive release of the excitatory neurotransmitter glutamate and subsequent activation of NMDA receptor and AMPA receptors. The persistent and extreme stimulation of neuronal activity yields high levels of reactive oxygen species (ROS), and eventually leads to cell degeneration and death. Excitotoxicity is considered to be one of the main underlying drivers behind neurodegeneration, brain injury and age-related cognitive decline.
EMF in the ELF range can indeed influence glutamate concentrations at the neuronal synapse, producing 40% higher or 35% lower concentrations depending on the frequency, intensity, and duration of exposure [10].
A variety of proteins involved in the glutamate receptor signalling pathway in the hippocampus were also significantly elevated after prolonged exposure to radio-frequency EMFs (900-1200MHz) [11].
RF-EMF exposure also enhanced glutamate-induced cytotoxicity and cell death in mouse hippocampal HT22 cells, and the introduction of an antioxidant (NAC) provided complete protection against this [12]. Together, these results suggest that excess glutamate may indeed play a key role in EMF-induced brain damage, and this is an important point to remember. However, there are also other neurotransmitters which are affected through EMF exposure, albeit in different ways.
Hippocampal acetylcholine release was decreased by 40% after exposure to 2.45 GHz radiation a 4mW/cm2, whereas it was unaffected at lower intensity [13]. Additionally, one hour of 800 MHz also produced no changes in acetylcholine, but 14 hours induced a 43% decrease. This suggests that changes in the cholinergic system of this brain region can be altered in different ways depending on the power output and frequency of radiation.
Another study showed significant disturbances in norepinephrine (noradrenaline), dopamine, and 5-hydroxytrypophan, with the authors concluding that these changes “may underlie many of the adverse effects reported after EMR including memory, learning, and stress.” [14]
Alterations in N-methyl-d-aspartate (NMDA) and GABAA receptors, along with dopamine transporters, were also found in rat brains exposed to 15 minutes of 900-MHz radiation emitted from a GSM device. That same study identified a strong glial reaction in the striatium region of the brain, potentially suggestive of neuronal damage [15].
A separate experiment showed that other areas of the brain including the cortex, hippocampus, and basal ganglia exhibited significant neuronal damage after rats were exposed to a GSM device for just 2 hours [16]. Numerous other studies have demonstrated neurobehavioral impairments [17], increase blood-brain barrier permeability [18], brain structure alterations [19] and cognitive deficits [20].
To make matters worse, 5 hours per day of 835 MHz RF-EMF caused great damage to the myelin sheath which resulted in demyelination. Just for your reference, myelin is the protective fatty coating which lines neurons, and is essentially responsible for facilitating the passage of signals from one neuron to the next. Without myelin, nerve signals cease and the nervous system as a whole is unable to effectively coordinate activity. Progressive demyelination has been identified in several neurodegenerative conditions, one of which is Multiple Sclerosis [21].
As I highlighted previously, the common offender in EMF-related tissue and cellular damage is disturbed redox balance and oxidative stress. The body’s main defence against this is the endogenous antioxidant system, which is tasked with protecting cells from the destructive effects of reactive oxygen species. When this innate system is unable to effectively manage oxidative injury, this is referred to as “oxidative stress”. Reduced antioxidant capacity in the context of elevated ROS can quite easily lead to nerce cell death and tissue destruction, so it is crucially important to maintain a healthy balance between oxidants and reducing agents.
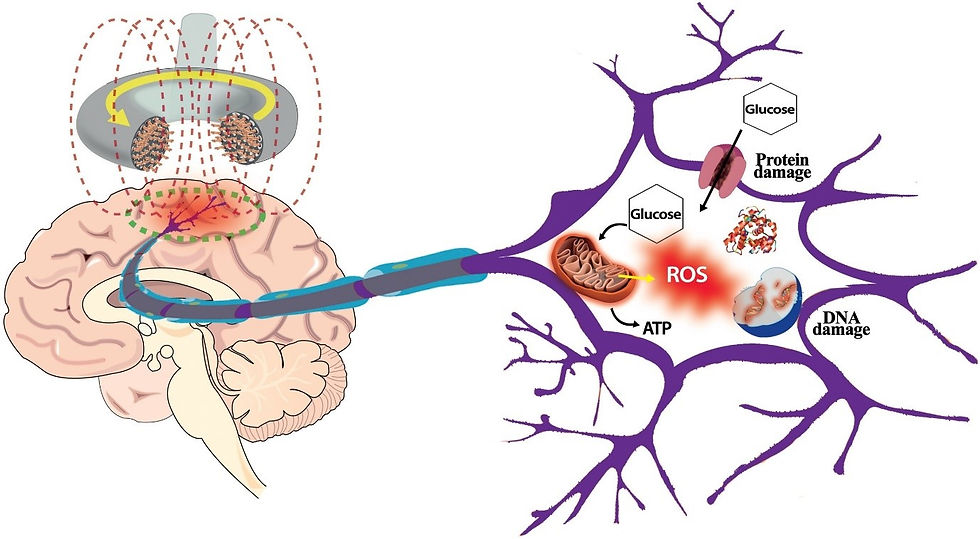
Kivrak E, Yurt K, Kaplan A, Alkan I, Altun G. Effects of electromagnetic fields exposure on the antioxidant defense system. Journal of Microscopy and Ultrastructure. 2017;5(4):167. doi:10.1016/j.jmau.2017.07.003
Mitochondria, as the main metabolic hub responsible for energy synthesis in the cells, are known to be the main source of intracellular ROS. ROS generation occurs as a normal byproduct of energy metabolism and plays a variety of important signalling roles, but can increase to pathological levels when mitochondria are stressed, damaged and dysfunctional. And like I said before, perhaps one of the primary mechanisms behind EMF-induced damage is mitochondrial dysfunction.
Mitochondria appear to be quite susceptible to injury from EMFs. Long-term, low dose cumulative MW-EMF exposure leads to structural damage which includes swelling, cavitation, and broken cristae of mitochondria in the hippocampus and cortex [22].
Significant reductions in ATP synthesis by hippocampal mitochondria were found in rats exposed to pulsed MW [23], whilst succinate dehydrogenase, a key enzyme in energy metabolism, also demonstrated much lower activity. Another mitochondrial enzyme called Cytochrome C oxidase (COX) is also affected. COX sits at the last step of ATP synthesis, where it is tasked with transferring electrons to oxygen to produce H2O and ATP. After irradiation with MW-EMF, COX enzyme activity, COX mRNA, and COX I protein were significantly reduced [23].
The exact ways by which EMFs destroy mitochondrial integrity are not yet fully not understood, although it seems to include the following:
EMF can lead to abnormal expression of genes encoding proteins in the respiratory chain, producing errors in energy metabolism [24]
EMF can damage the mitochondrial membrane though altering molecular rotation, vibration and collision frequency to produce changes in membrane structure [25]
The activation of NADH oxidase by EMF mediates an increase in ROS, which likely targets the mitochondrial membrane, disrupts mitochondrial efficiency, and overwhelms the cell. Dysfunctional mitochondria then go on to produce more ROS in a vicious cycle. ROS may also damage mitochondrial and nuclear DNA, create DNA strand breakages and mutations, and reduce energy synthesis capacity [26].
Calcium efflux or “overload” can occur, where high concentrations of calcium ions flood into the cell through voltage gated calcium channels. This can activate the mitochondrial permeability transition pore, resulting in swelling, fragmentation, and even cell death [27].
The resulting consequence is that cells lose the ability to make energy in an efficient, or “clean” way. Mitochondria begin to spew out excessive ROS. Likewise, the ROS generated via other sources can quite easily overwhelm our endogenous protective systems to produce a catastrophic state of oxidative stress.
Pineal release of melatonin, the brain’s primary antioxidant and radical scavenger, is substantially reduced under exposure to specific frequencies of radiation. Unfortunately, low melatonin is a key risk factor for the development of neurological dysfunction, neurodegeneration, and other pathologies including cancer of various kinds. Furthermore, key intracellular antioxidants including glutathione, catalase, and superoxide dismutase are also deteriorated through ELF-EMF exposure at 60Hz [28].
EMF emitted by mobile devices was found to deplete key glutathione biomarkers including GSH (reduce glutathione), glutathione peroxidase, glutathione reductase and glutathione s-transferase in rat brains. Malondialdehyde, a marker of lipid peroxidation, was also greatly elevated [29].
A different study on guinea pig brain tissue also showed a reduction in GSH, catalase, and elevations in malondialdehyde [30]. Together, these biochemical parameters are consistent with a state of excessive oxidative stress in the brain cells.
It is important that you know there are many other studies demonstrating effects consistent with this. Namely, that chronic EMF exposure takes a massive toll on the endogenous antioxidants.
To summarize so far:
EHS/EMF-Sensitivity is a genuine physiological condition characterised by altered biomarkers associated with oxidative stress
EMF exposure alters levels of neurotransmitters including acetylcholine, dopamine, norepinephrine, and glutamate. Excess glutamate may produce excitotoxicity, which can lead to cell death.
EMF exposure produces damage to neurons and brain tissue via increased generation of ROS
EMF exposures can damage mitochondria in several ways to cause mitochondrial dysfunction, which further enhances ROS production and reduces ATP
The oxidative burden associated with chronic EMF is associated with reduced levels of antioxidants including melatonin, glutathione, and other antioxidant enzymes.
With the above in mind, I hope that you can appreciate the genuine dangers posed by exposures to these electronic devices and other sources of electromagnetic radiation. Whilst I believe that the best protection against this is strict avoidance, it is frankly not possible in our modern world. Sleep and circadian hygiene, intelligent movement, stress-management and a nutrient-dense diet are "no-brainers".
However, these changes are not always successful. The next best solution is to devise strategies to aid our cell’s ability to mitigate some of the damage through supporting mitochondria and reducing the oxidative burden. As we will examine next, thiamine possesses many of the characteristics which make it a useful candidate for supporting neurological health and protecting cells against some of the detrimental effects exerted by EMFs.
EMF & Thiamine connection
First, I should make clear that no studies (to my knowledge) that have been done on the direct actions of thiamine in relation to EMF. However, my clinical experience with people who have EHS coupled with the mechanistic data justifies the use of this nutrient in my opinion. Thiamine’s benefits for the brain and neurological system are well-established, and a thiamine deficiency is known to primarily manifest as neurological dysfunction in many cases.
The mitochondrial dysfunction and oxidative stress induced by EMF no doubt increase thiamine requirements. On the other hand, an oxidative environment is also thoroughly detrimental to thiamine homeostasis. Not only does oxidation increase the need for thiamine in the brain, but oxidative stress also disturbs thiamine homeostasis and negatively influences the enzymes which use thiamine as a cofactor.
Protecting cells from the destructive consequences of excessive oxidation requires a continual supply of energy. The active form of thiamine (TPP) plays a coenzyme role for multiple enzymes required for the generation of this energy in the form of ATP. The pyruvate dehydrogenase complex (PDHC), sitting at the entry point to oxidative metabolism of glucose, requires thiamine as a cofactor. The rate of glucose metabolism has been shown to rapidly increase in response to EMF in brain regions closest the antennae [31]. Alone, this naturally increases thiamine turnover.
Alpha-ketoglutarate dehydrogenase (KGDH) is another thiamine-dependent mitochondrial enzyme complex involved in the TCA cycle. Of all of the enzymes involved in energy metabolism, KGDH is one of the most sensitive to oxidative stress [32].
Several biological effects associated with EMFs have been shown to inactivate this enzyme, including elevations of nitric oxide and peroxynitrate [33], along with high intracellular calcium concentrations [34]. Since KGDH is one of the rate-limiting steps in mitochondrial oxidative metabolism, an inhibition of this enzyme can have severe downstream consequences, slowing down the rate of energy metabolism and producing an ATP deficit. For this reason, the downregulation/inactivation of KGDH is thought to play an integral part in the development of neurodegenerative processes.
However, this enzyme complex is not only necessary for energy turnover, but also plays a dual role in protecting neurons from the damaging effects of excess glutamate. Through anaplerosis, glutamate is fed into the TCA cycle as the energy intermediate alpha-ketoglutarate. In the context of low KGDH activity, a “backlog” of alpha-ketoglutarate results in the accumulation of glutamate. In line with this, evidence shows that thiamine deficiency increases extracellular glutamate concentrations [35] to induce neurotoxic lesions in the brain [36]. A deficiency also reduces glutamate uptake in neurons [37], which ordinarily helps to reduce extracellular glutamate concentrations, and this occurs likely through downregulation of GLT-1 and GLAST glutamate transporters [38].
But the benefits of thiamine in protecting against glutamate excitotoxicity and mitochondrial dysfunction are NOT simply limited to those who are thiamine deficient. This point was wonderfully illustrated in a study on traumatic brain injury in rats [39]. Researchers found that pre-treatment with high dose thiamine (400mg/KG) completely prevented oxidative stress-induced inactivation of alpha-ketoglutarate dehydrogenase, reduced glutamate concentrations, and increased the rate at which it was fed into the TCA cycle, thereby maintaining good mitochondrial function.
In other words, despite having normal thiamine status, doses higher than nutritional requirement were necessary to protect mitochondrial function from the damage caused by traumatic brain injury. Recall the fMRI study above, where researchers likened the results of an EHS patient to that of traumatic brain injury? The neurochemical changes associated with TBI are similar in many ways to chronic EMF exposure – glutamate excitotoxicity, chronic oxidative stress, mitochondrial dysfunction and neuroinflammation. This research showed that high-dose thiamine essential kick-started the activity of specific enzymes to “bypass” the stressor and restore normal function.
Thiamine’s potential role in supporting brain cells against damage by EMF also relates to another thiamine-dependent enzyme called transketolase, which acts as a “bridge” in the pentose phosphate pathway (PPP). Transketolase is thought to be a “redox-sensitive regulatory mechanism to increase flux through the PPP in times of oxidative stress” [40]. When excessive oxidation occurs in cells, this inevitably raises the requirement for glutathione to counteract the damage.
Cells regenerate glutathione by using the NADPH generated in the PPP. Hence, increased oxidation means increased transketolase activity, which, by definition, also translates to an increased thiamine requirement. And not only does pathway this supply reducing power to regenerate glutathione, but also diverts resources towards fatty acid synthesis required for maintaining the myelin sheath. This is especially important in the context of EMF-induced myelin sheath degeneration and demyelination.
As we examined earlier, low glutathione and other intracellular antioxidants are characteristic biomarkers of EHS/EMF-sensitivity. In fact, EMF exposure alone is enough to deplete brain levels of these antioxidants and render cells susceptible to oxidative damage.
Again, thiamine has been used in the research to prevent this antioxidant deficit and protect cells from toxicity. Although there are no studies looking at thiamine in the context of EMF specifically, active thiamine (TPP) has been used successfully for toxic exposures which produce similar neurochemical changes. High dose thiamine prevented oxidative damage caused by cisplatin in both liver [41] and brain [42], reducing markers of lipid peroxidation, DNA damage, and increasing levels of key antioxidants - reduced glutathione and superoxide dismutase.
In brain methotrexate toxicity, TPP prevented the increase in lipid peroxidation and maintained levels of reduced glutathione, glutathione peroxidase, and superoxide dismutase [43]. A lipid-soluble disulphide derivative of thiamine called sulbutiamine was shown to have a similar protective effect on glutathione and significantly reduced reactive oxygen species in retinol ganglion cells [44].
Thiamine as an antioxidant
However, these anti-oxidative effects are likely not solely due to thiamine’s role as a cofactor for transketolase. Rather, one possible function of the thiamine molecule is a site-directed antioxidant. Thiamine can exert antioxidant effects on ascorbic acid [45], aldehydes and polyphenols [46], and scavenge superoxide [47].
When complexed with ascorbic acid, it was also shown to inhibit the oxidation of dopamine [48]. The suggested mechanism by which it directly quenches free radicals and hydroperoxides is by the transfer of two electrons from the NH2 group of the pyrimidine ring of the thiamine molecule [46].
Interestingly, thiamine can reverse forms of oxidative stress which are not directly related to its coenzyme function. Lipid peroxidation and glutathione reductase activity in cardiac hypertrophy were normalized with thiamine supplementation [49], along with free radical oxidation of oleic acid in rat liver microsomes [46].
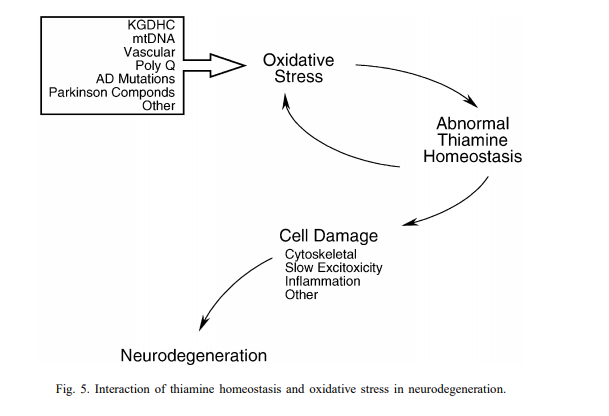
Gibson G. Interactions of oxidative stress with thiamine homeostasis promote neurodegeneration. Neurochemistry International. 2002;40(6):493-504. doi:10.1016/s0197-0186(01)00120-6
So it would seem that thiamine might be useful in counteracting many of the specific physiological consequences of EMF exposure. When considering the different types of thiamine which might come in handy in this context, it is important to use a form which can saturate the brain.
As an aside, there are several other nutrients which have been proven to support oxidation in the brain.
These include selenium, melatonin, and vitamin E. However, there are multiple nutrients which have received attention in the research community and show excellent promise for protecting cell metabolism. Many studies have demonstrated protective effects on melatonin specifically in relation to EMF damage. Melatonin was shown to reduce lipid peroxidation and boost glutathione peroxidase and reduced glutathione after wifi exposure [50].
According to another study, melatonin protected hippocampal cells through reducing malondialdehyde and reversing the loss of glutathione peroxidase, SOD, and catalase [51]. Finally, both melatonin and omega 3 fatty acids protected against damage and cell death in the hippocampus [52]. Other research has also highlighted significant benefits from vitamin C [53], N-acetylcysteine, Vitamin E [54, 55], Selenium [56], zinc [57], and folate [58].
REFERENCES 1. Irigaray P, Caccamo D, Belpomme D. Oxidative stress in electrohypersensitivity self reporting patients: Results of a prospective in vivo investigation with comprehensive molecular analysis. International Journal of Molecular Medicine. July 2018. doi:10.3892/ijmm.2018.3774 2. DeLuca C, Chung Sheun Thai J, Raskovic D, et al. Metabolic and Genetic Screening of Electromagnetic Hypersensitive Subjects as a Feasible Tool for Diagnostics and Intervention. Mediators of Inflammation. 2014;2014:1-14. doi:10.1155/2014/924184 3. LandgrebeM, Frick U, Hauser S, et al. Cognitive and neurobiological alterations in electromagnetic hypersensitive patients: results of a case-control study. Psychological Medicine. 2008;38(12):1781-1791. doi:10.1017/s0033291708003097 4. HeuserG, Heuser SA. Functional brain MRI in patients complaining of electrohypersensitivity after long term exposure to electromagnetic fields. Reviews on Environmental Health. 2017;32(3). doi:10.1515/reveh-2017-0014 5. Electromagnetic Hypersensitivity: Evidence for a Novel Neurological Syndrome. International Journal of Neuroscience.https://www.tandfonline.com/doi/abs/10.3109/00207454.2011.608139. Published2011. Accessed May 16, 2020. 6. Ghezel-Ahmadi D, Engel A, Weidemann J, et al. Heavy metal exposure in patients suffering from electromagnetic hypersensitivity. The Science of the total environment.2010;408(4):774-778. doi:10.1016/j.scitotenv.2009.11.023 7. Fujii Y. Sensation of Balance Dysregulation Caused/Aggravated by a Collection of Electromagnetic Waves in a Dental Implant. Open Journal of Antennas and Propagation. 2014;02(03):29-35. doi:10.4236/ojapr.2014.23004 8. Jain A, Hasan S, Ganapathy D. Effect of radiation from mobile devices on mercury leaching in dental practice Effect of radiation from mobile devices on mercury leaching in dental practice. Drug Invention Today |. 2018;10. https://www.researchgate.net/profile/Ashish_Jain52/publication/330354129_Effect_of_radiation_from_mobile_devices_on_mercury_leaching_in_dental_practice/links/5c5ec42c92851c48a9c4e997/Effect-of-radiation-from-mobile-devices-on-mercury-leaching-in-dental-practice.pdf.Accessed May 16, 2020. 9. Mortazavi SMJ, Daiee E, Yazdi A, et al. Mercury release from dental amalgam restorationsafter magnetic resonance imaging and following mobile phone use. Pakistanjournal of biological sciences : PJBS. 2008;11(8):1142-1146.doi:10.3923/pjbs.2008.1142.1146 10. Masoudian N, Riazi GH, Afrasiabi A, et al. Variations of Glutamate Concentration Within Synaptic Cleft in the Presence of Electromagnetic Fields: An Artificial Neural Networks Study. Neurochemical Research. 2015;40(4):629-642.doi:10.1007/s11064-014-1509-6 11. Effects of acute and chronic exposure to both 900 MHz and 2100 MHz electromagnetic radiation on glutamate receptor signaling pathway. International Journal of Radiation Biology.https://www.tandfonline.com/doi/abs/10.1080/09553002.2017.1337279?journalCode=irab20.Published 2017. Accessed May 16, 2020. 12. Effects of radiofrequency field exposure on glutamate-induced oxidative stress in mouse hippocampal HT22 cells. International Journal of Radiation Biology.https://www.tandfonline.com/doi/abs/10.1080/09553002.2017.1237058?journalCode=irab20.Published 2017. Accessed May 16, 2020. 13. Testylier G, Tonduli L, Malabiau R, Debouzy JC. Effects of exposure to low level radiofrequency fields on acetylcholine release in hippocampus of freely moving rats. Bioelectromagnetics. 2002;23(4):249-255. doi:10.1002/bem.10008 14. Aboulezz H, Ezz A, Khadrawy Y, Radwan N. The Effect of Pulsed Electromagnetic Radiation from Mobile Phone on the Levels of Monoamine Neurotransmitters in Four Different Areas of Rat Brain A Study on the Influence of Mobile Phone Radiation on Brain EEG and Neurotransmission. View Project New Approach in Novel Drug Discovery View Project.; 2014.https://www.researchgate.net/profile/Heba_Aboulezz_or_Aboul_Ezz/publication/249321687_The_effect_of_pulsed_electromagnetic_radiation_from_mobile_phone_on_the_levels_of_monoamine_neurotransmitters_in_four_different_areas_of_rat_brain/links/02e7e51f7856781a33000000/The-effect-of-pulsed-electromagnetic-radiation-from-mobile-phone-on-the-levels-of-monoamine-neurotransmitters-in-four-different-areas-of-rat-brain.pdf.Accessed May 16, 2020. 15. MAUSSETBONNEFONTA, HIRBEC H, BONNEFONT X, PRIVAT A, VIGNON J, DESEZE R. Acute exposure to GSM900-MHz electromagnetic fields induces glial reactivity and biochemical modifications in the rat brain. Neurobiology of Disease.2004;17(3):445-454. doi:10.1016/j.nbd.2004.07.004 16. Nerve cell damage in mammalian brain after exposure to microwaves from GSM mobile phones. Environmental Health Perspectives. https://ehp.niehs.nih.gov/doi/10.1289/ehp.6039. Published 2018. Accessed May16, 2020. 17. Roggeveen S, van Os J, Lousberg R. Does the Brain Detect 3G Mobile Phone Radiation Peaks? An Explorative In-Depth Analysis of an Experimental Study. Akiba S, ed. PLOSONE. 2015;10(5):e0125390. doi:10.1371/journal.pone.0125390 18. Sırav B, Seyhan N. Effects of GSM modulated radio-frequency electromagnetic radiation on permeability of blood-brain barrier in male & female rats. Journal of chemical neuroanatomy. 2016;75(Pt B):123-127.doi:10.1016/j.jchemneu.2015.12.010 19. Effects of mobile phone radiation (900 MHz radiofrequency) on structure and functions of rat brain. Neurological Research. https://www.tandfonline.com/doi/full/10.1179/1743132814Y.0000000392. Published2014. Accessed May 16, 2020. 20. Megha K, Deshmukh PS, Ravi AK, Tripathi AK, Abegaonkar MP, Banerjee BD. Effect of Low-Intensity Microwave Radiation on Monoamine Neurotransmitters and Their Key Regulating Enzymes in Rat Brain. Cell biochemistry and biophysics.2015;73(1):93-100. doi:10.1007/s12013-015-0576-x 21. Kim JH, Yu D-H, Huh YH, Lee EH, Kim H-G, Kim HR. Long-term exposure to 835 MHz RF-EMF induces hyperactivity, autophagy and demyelination in the cortical neurons of mice. Scientific Reports. 2017;7(1). doi:10.1038/srep41129 22. Europe PMC. Europe PMC. Europepmc.org. https://europepmc.org/article/med/15130438.Published 2019. Accessed May 16, 2020. 23. INIS Repository Search - Single Result. Iaea.org.https://inis.iaea.org/search/searchsinglerecord.aspx?recordsFor=SingleRecord&RN=40060307.Published 2020. Accessed May 16, 2020. 24. Effects of AduoLa Fuzhenglin on changes of cytochrome c oxidase in rats′ hippocampus after microwave exposure--《Military Medical Sciences》2011年05期.Cnki.com.cn. http://en.cnki.com.cn/Article_en/CJFDTotal-JSYX201105004.htm.Published 2011. Accessed May 16, 2020. 25. WANG,Y.A study on thermal efficiency and non-thermal efficiency of microwave. Liaoning Chem Ind. 2011;35:167-169. https://ci.nii.ac.jp/naid/10029657981/. Accessed May 16, 2020. 26. Yahyazadeh A, Deniz ÖG, Kaplan AA, Altun G, Yurt KK, Davis D. The genomic effects of cellphone exposure on the reproductive system. Environmental research.2018;167:684-693. doi:10.1016/j.envres.2018.05.017 27. Petronilli V, Penzo D, Scorrano L, Bernardi P, Di Lisa F. The Mitochondrial Permeability Transition, Release of Cytochrome c and Cell Death. Journal of Biological Chemistry. 2000;276(15):12030-12034.doi:10.1074/jbc.m010604200 28. LeeB-C, Johng H-M, Lim J-K, et al. Effects of extremely low frequency magnetic field on the antioxidant defense system in mouse brain: a chemiluminescence study. Journal of photochemistry and photobiology B, Biology.2004;73(1-2):43-48. doi:10.1016/j.jphotobiol.2003.10.003 29. SharmaS, Shukla S. Effect of electromagnetic radiation on redox status, acetylcholine esterase activity and cellular damage contributing to the diminution of the brain working memory in rats. Journal of Chemical Neuroanatomy.2020;106:101784. doi:10.1016/j.jchemneu.2020.101784 30. Meral I, Mert H, Mert N, et al. Effects of 900-MHz electromagnetic field emitted from cellular phone on brain oxidative stress and some vitamin levels of Guinea pigs. Brain Research. 2007;1169:120-124.doi:10.1016/j.brainres.2007.07.015 31. Volkow ND. Effects of Cell Phone Radiofrequency Signal Exposure on Brain Glucose Metabolism. JAMA. 2011;305(8):808. doi:10.1001/jama.2011.186 32. TretterL, Adam-Vizi V. Alpha-ketoglutarate dehydrogenase: a target and generator ofoxidative stress. Philosophical Transactions of the Royal Society B:Biological Sciences. 2005;360(1464):2335-2345. doi:10.1098/rstb.2005.1764 33. Andersson U, Leighton B, Young ME, Blomstrand E, Newsholme EA. Inactivation of aconitase and oxoglutarate dehydrogenase in skeletal muscle in vitro by superoxide anions and/or nitric oxide. Biochemical and biophysical research communications.1998;249(2):512-516. doi:10.1006/bbrc.1998.9171 34. Lai JC, Cooper AJ. Brain alpha-ketoglutarate dehydrogenase complex: kinetic properties, regional distribution, and effects of inhibitors. Journal of neurochemistry.1986;47(5):1376-1386. doi:10.1111/j.1471-4159.1986.tb00768.x 35. Langlais PJ, Zhang SX. Extracellular glutamate is increased in thalamus during thiamine deficiency-induced lesions and is blocked by MK-801. Journal of neurochemistry. 1993;61(6):2175-2182.doi:10.1111/j.1471-4159.1993.tb07457.x 36. Hazell AS, Butterworth RF, Hakim AM. Cerebral vulnerability is associated with selective increase in extracellular glutamate concentration in experimental thiamine deficiency. Journal of neurochemistry. 1993;61(3):1155-1158.doi:10.1111/j.1471-4159.1993.tb03635.x 37. Europe PMC. Europe PMC. Europepmc.org. https://europepmc.org/article/med/16687165.Published 2019. Accessed May 16, 2020. 38. Hazell AS, Rao KV, Danbolt NC, Pow DV, Butterworth RF. Selective down-regulation of the astrocyte glutamate transporters GLT-1 and GLAST within the medial thalamusin experimental Wernicke’s encephalopathy. Journal of neurochemistry.2001;78(3):560-568. doi:10.1046/j.1471-4159.2001.00436.x 39. Mkrtchyan GV, Üçal M, Müllebner A, et al. Thiamine preserves mitochondrial function in a rat model of traumatic brain injury, preventing inactivation of the2-oxoglutarate dehydrogenase complex. Biochimica et Biophysica Acta (BBA) -Bioenergetics. 2018;1859(9):925-931. doi:10.1016/j.bbabio.2018.05.005 40. Wilkinson HC, Dalby PA. Novel insights into transketolase activation by cofactor binding identifies two native species subpopulations. Scientific Reports.2019;9(1). doi:10.1038/s41598-019-52647-y 41. Turan MI, Siltelioglu Turan I, Mammadov R, Altınkaynak K, Kisaoglu A. The effect of thiamine and thiamine pyrophosphate on oxidative liver damage induced in rats with cisplatin. BioMed research international. 2013;2013:783809.doi:10.1155/2013/783809 42. Turan MI, Cayir A, Cetin N, Suleyman H, Siltelioglu Turan I, Tan H. An investigation of the effect of thiamine pyrophosphate on cisplatin-induced oxidative stress and DNA damage in rat brain tissue compared with thiamine: thiamine and thiamine pyrophosphate effects on cisplatin neurotoxicity. Human &experimental toxicology. 2014;33(1):14-21. doi:10.1177/0960327113485251 43. TURANM, Cetin N, Siltelioglu Turan, Isil, ÖZGERİŞ F, Suleyman H. Effects of Thiamine and Thiamine Pyrophosphate on Oxidative Stress By Methotrexate in The Rat Brain. Ebyu.edu.tr. 2013. doi:d3b61462-1862-4b8f-b4e2-ba966de3508c 44. Majid ASA, Yin ZQ, Ji D. Sulphur antioxidants inhibit oxidative stress induced retinal ganglion cell death by scavenging reactive oxygen species but influence nuclear factor (erythroid-derived 2)-like 2 signalling pathway differently. Biological& pharmaceutical bulletin. 2013;36(7):1095-1110.doi:10.1248/bpb.b13-00023 45. CAB Direct. Cabdirect.org.https://www.cabdirect.org/cabdirect/abstract/19511404784. Published 2020.Accessed May 16, 2020. 46. Lukienko PI, Mel’nichenko NG, Zverinskii IV, Zabrodskaya SV. Bulletin of Experimental Biology and Medicine. 2000;130(9):874-876. doi:10.1023/a:1015318413076 47. Jung IL, Kim IG. Thiamine protects against paraquat-induced damage: scavenging activity of reactive oxygen species. Environmental toxicology and pharmacology. 2003;15(1):19-26. doi:10.1016/j.etap.2003.08.001 48. Florence TM, Stauber JL. Manganese catalysis of dopamine oxidation. Science of The Total Environment. 1989;78:233-240. doi:10.1016/0048-9697(89)90036-3 49. Tolstykh OI, Khmelevskiĭ IV. The role of alpha-tocopherol and thiamine in the correction of lipid peroxidation in compensatory myocardial hypertrophy. Voprosypitaniia. 1991;(3):38-42. https://www.ncbi.nlm.nih.gov/pubmed/1833883.Accessed May 16, 2020. 50. Nazıroğlu M, Kahya M, Tök Ö, Doğan S, Tök L. Effects of melatonin on Wi-Fi-induced oxidative stress in lens of rats. Indian Journal of Ophthalmology.2014;62(1):12. doi:10.4103/0301-4738.126166 51. Memduh Kerman, Nilgun Senol. 3rd International Conference on Molecular Biology and Stem Cells. Biomedical Research. 2019;23(1).https://www.alliedacademies.org/articles/oxidative-stress-in-hippocampus-induced-by-900-mhz-electromagnetic-field-emitting-mobile-phone-protection-by-melatonin.html.Accessed May 16, 2020. 52. Altun G, Kaplan S, Deniz O, et al. Protective effects of melatonin and omega-3 on the hippocampus and the cerebellum of adult Wistar albino rats exposed to electromagnetic fields. Journal of Microscopy and Ultrastructure.2017;5(4):230. doi:10.1016/j.jmau.2017.05.006 53. Oral B, Guney M, Ozguner F, et al. Endometrial apoptosis induced by a 900-MHz mobile phone: Preventive effects of vitamins E and C. Advances in Therapy.2006;23(6):957-973. doi:10.1007/bf02850217 54. Ghanbari A, Shabani K, Mohammad Nejad D. Protective Effects of Vitamin E Consumption against 3MT Electromagnetic Field Effects on Oxidative Parameters in Substantia Nigra in Rats. Basic and Clinical Neuroscience Journal. 2016;7(4).doi:10.15412/j.bcn.03070404 55. Protective effects of vitamin E against electromagnetic radiation from cell phones in pregnant and fetal rats’ brain tissues--《Journal of Shandong University(Health Sciences)》2011年09期. Cnki.com.cn.http://en.cnki.com.cn/Article_en/CJFDTotal-SDYB201109009.htm. Published 2011.Accessed May 16, 2020. 56. Pastacı Özsobacı N, Düzgün Ergün D, Durmuş S, et al. Selenium supplementation ameliorates electromagnetic field-induced oxidative stress in the HEK293 cells. Journal of trace elements in medicine and biology : organ of the Society for Minerals and Trace Elements (GMS). 2018;50:572-579.doi:10.1016/j.jtemb.2018.04.008 57. Bediz CS, Baltaci AK, Mogulkoc R, Oztekin E. Zinc supplementation ameliorates electromagnetic field-induced lipid peroxidation in the rat brain. The Tohoku journal of experimental medicine. 2006;208(2):133-140.doi:10.1620/tjem.208.133 58. Altunkaynak B, Kivrak E, Alkan I, Yurt K, Kocaman A, Onger M. Effects of 900-MHz radiation on the hippocampus and cerebellum of adult rats and attenuation of such effects by folic acid and Boswellia sacra. Journal of Microscopy and Ultrastructure.2017;5(4):216. doi:10.1016/j.jmau.2017.09.003
Comments